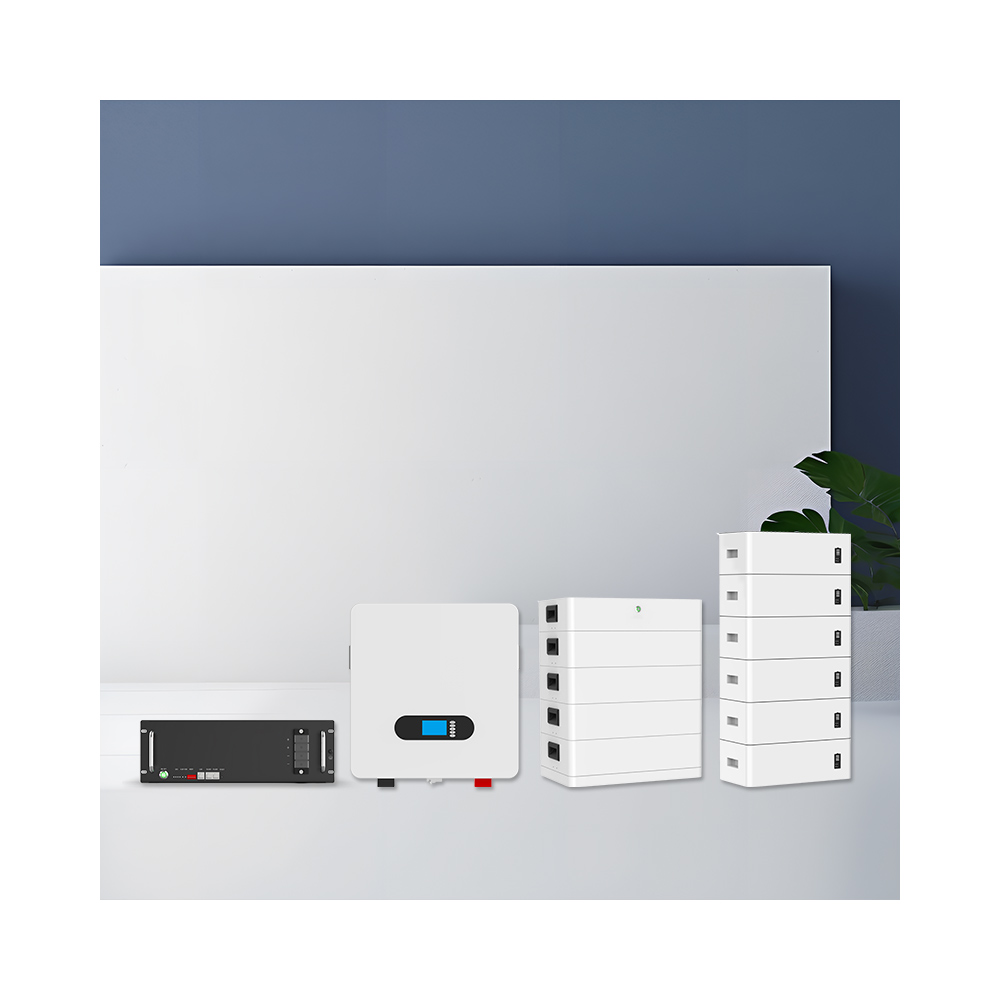
Lithium batteries have become an integral part of modern technology, owing to their high energy density and long cycle life. These batteries come in various chemistries, each tailored for specific applications. In this article, we will explore six types of lithium batteries, discussing their unique characteristics, advantages, and common uses to provide a comprehensive understanding of the options available in the market today.
What Is A Lithium-Ion Battery?
A Lithium-ion (Li-ion) battery is a type of rechargeable battery technology that utilizes lithium ions as the primary component of its electrochemistry. The movement of lithium ions occurs between the negative electrode (anode) and the positive electrode (cathode) during charging and discharging cycles. Li-ion batteries are known for their high energy density, meaning they can store a significant amount of energy relative to their weight.
The structure of Li-ion batteries allows them to maintain a steady voltage output until their capacity is nearly exhausted, which contributes to the reliability of the power they provide. Unlike some other rechargeable batteries, these do not suffer from the memory effect—a phenomenon where batteries lose their maximum energy capacity if they are repeatedly recharged after being only partially discharged.
In terms of longevity, Li-ion batteries also offer an advantageous cycle life, which refers to the number of complete charge and discharge cycles they can undergo before their capacity drops below a certain percentage of original capacity. Their self-discharge rate—how quickly they lose charge while idle—is relatively low compared to other battery types.
Furthermore, because lithium ions can move freely through the electrolyte with minimal resistance, this translates into an impressive rate capability in both charging (C-rate) and discharging phases. However, safety concerns are present due to risks associated with thermal runaway events—situations where excessive heat buildup can lead to ignition or explosion if not properly managed.
Form factors for Li-ion batteries vary widely, allowing for bespoke solutions that fit specific device requirements including but not limited to smartphones, laptops, electric vehicles, and large-scale energy storage systems. Overall, Li-ion technology provides a balance of performance attributes that have made it a widespread choice among various applications requiring portable power sources or storage solutions.
6 Types Of Lithium Batteries
Lithium batteries are renowned for their high energy density and ability to undergo numerous charging cycles before degrading, making them ideal for a variety of applications from mobile electronics to electric vehicles. Among the diverse types of lithium batteries, six primary chemistries have emerged as the most prevalent, each with its distinct characteristics and uses. These include Lithium Iron Phosphate (LiFePO4), Lithium Cobalt Oxide (LiCoO2), Lithium Manganese Oxide (LiMn2O4), Lithium Nickel Manganese Cobalt Oxide (LiNiMnCoO2 or NMC), Lithium Nickel Cobalt Aluminium Oxide (LiNiCoAlO2 or NCA), and Lithium Titanate (Li4Ti5O12 or LTO).
- Lithium Iron Phosphate (LiFePO4):
- Offers long cycle lives.
- Notable for safety features with a lower risk of thermal events.
- Employed in applications where longevity and reliability are crucial.
- Lithium Cobalt Oxide (LiCoO2):
- One of the earliest lithium-ion battery chemistries.
- Known for high specific energy, making it suitable for compact and lightweight consumer electronics.
- Lithium Manganese Oxide (LiMn2O4):
- Distinguished by a stable spinel structure, providing high thermal stability and improved safety.
- Lower specific energy compared to other variants.
- NMC (Lithium Nickel Manganese Cobalt Oxide):
- Offers a balanced approach with a good mix of specific energy, capacity, and power.
- Widely used in electric vehicle powertrains and other applications.
- Lithium Nickel Cobalt Aluminium Oxide (LiNiCoAlO2):
- Stands out for impressive specific power and energy densities.
- Favored in high-end consumer electronics and electric vehicles for longer run times per charge.
- Lithium Titanate (Li4Ti5O12):
- Charges exceptionally quickly.
- Offers one of the best cycle life performances.
- Lower intrinsic voltage reduces overall gravimetric energy density.
- Used in specialized areas where rapid charging or extreme cycle life is critical.
Understanding these six types allows us to appreciate the varied capabilities that exist within lithium battery technology, guiding users to choose the appropriate battery type based on their particular requirements such as voltage compatibility, capacity needs, expected lifecycle duration, required charge/discharge rates and operational safety standards.
Lithium Iron Phosphate (LiFePO₄) Batteries
Lithium Iron Phosphate, often abbreviated as LiFePO₄ or LFP, is one of the key types of lithium batteries known for its robustness and safety. The pros of using Lithium Iron Phosphate include a significantly lower risk of thermal runaway because of its stable chemical structure, thus enhancing safety. Additionally, these batteries offer an impressively long cycle life, often surpassing that of other lithium battery types. They exhibit high charge and discharge rates (C-rates), making them suitable for scenarios requiring rapid energy transfer.
Conversely, the cons are centered predominantly around their energy density; Lithium Iron Phosphate batteries typically deliver less specific energy (or capacity) compared to some alternative lithium-based chemistries. This translates into larger and heavier batteries to provide the same amount of energy as other types such as Lithium Cobalt Oxide.
When analyzing features specific to Lithium Iron Phosphate batteries:
- Voltages: Each cell has a nominal voltage generally around 3.2V.
- Specific Energy (Capacity): Ranging from about 90-120 Wh/kg, they provide less energy per unit weight than some alternatives.
- Charge (C-Rate): These can sustain high charge rates up to 1C or more.
- Discharge (C-Rate): LFP batteries maintain high discharge rates without significant impact on battery life.
- Cycle Life: They commonly provide over 2000 cycles with minimal degradation, making them extremely durable.
- Thermal Runaway: The probability is significantly reduced due to the inherent stability of the phosphate-based cathode.
Applications suited for Lithian Iron Phosphate batteries typically involve instances where safety and longevity outweigh the need for compact energy storage solutions—examples include electric vehicles that prioritize range consistency over time and various stationary storage systems leveraging renewable energy sources.
Pros Of Lithium Iron Phosphate and Cons Of Lithium Iron Phosphate
Pros of Lithium Iron Phosphate (LiFePO4) | Cons of Lithium Iron Phosphate (LiFePO4) |
---|---|
Robust Safety Profile: Thermal stability reduces the risk of thermal runaway. | Lower Energy Density: Less energy stored per unit of weight or volume. |
Long Cycle Life: Often exceeds 2000 cycles with minimal degradation. | Lower Voltage: Typically around 3.2 volts per cell, requiring more cells. |
Cost-Effective Long-Term: Durability and lower replacement frequency. | Higher Initial Costs: Manufacturing costs are comparatively higher. |
Ample Specific Energy: Ideal for various uses from electric vehicles to storage. | Reduced Power in Cold: Performance may decrease in low-temperature environments. |
Eco-Friendly: Lacks cobalt, offering a more sustainable alternative. | Long Cycle Life Challenges: May be less relevant in rapidly evolving technologies. |
Low Self-Discharge Rate: Preserves stored power effectively over time. | Limited Market Availability: Dominance of other chemistries limits variety. |
This table summarizes the pros and cons of Lithium Iron Phosphate (LiFePO4) batteries, highlighting their safety, cycle life, cost-effectiveness, energy density, and other factors that impact their suitability for various applications.
Features Of Lithium Iron Phosphate
Lithium Iron Phosphate batteries, often known by the acronym LFP or LiFePO4, are renowned for their robustness and safety. Voltages for these batteries typically peak at 3.2 volts per cell, which contributes to a stable and consistent power delivery during operation. In terms of specific energy (capacity), LFP batteries usually deliver between 90-120 watt-hours per kilogram, making them less dense in comparison to other lithium variants but sufficient for a myriad of applications.
The charge (C-Rate) and discharge rates of Lithium Iron Phosphate batteries are also exemplary owing to their stable chemistry. They can frequently handle high charge rates, commonly up to 1C (a charge rate equal to the battery’s capacity over one hour), enabling quick recharging relative to other chemistries. Discharging can be sustained at high rates as well, which makes these batteries particularly useful in applications requiring large bursts of power.
When discussing cycle life, LFP stands out because it can endure several thousand cycles before significant degradation occurs—often cited numbers exceed 2000 full charge-discharge cycles. This longevity is a definitive advantage as it translates into a longer lifespan.
Another critical feature of the Lithium Iron Phosphate battery is its resistance to thermal runaway, an event that leads to overheating and potential thermal instability in lithium batteries. The phosphate-based cathode material used in LFP provides a strong structure that withstands extreme temperatures better than other lithium compounds.
Regarding their use cases or applications, LFP batteries are ideal for scenarios where weight is not the primary concern but where longevity and stability take precedence. They are favored in stationary energy storage systems like those used with solar panels or backup power supplies, electric vehicles such as scooters and golf carts, and numerous industrial applications due to their dependable lifecycle performance and impressive safety profile.
Lithium Cobalt Oxide
Lithium Cobalt Oxide, often referred to by its chemical designation LiCoO2, is a type of lithium battery that offers distinct advantages and considerations. This chemistry is recognized for its high specific energy, or capacity, which makes it particularly valuable in applications where space and weight are at a premium such as in mobile phones and laptops.
The Pros of Lithium Cobalt Oxide batteries include their notably high energy density which allows for longer usage between charges, making them ideal for compact, energy-intensive devices. Additionally, they have a stable charge retention capability that contributes to their reliability in consumer electronics.
However, there are Cons to consider with Lithium Cobalt Oxide batteries. They typically have a relatively short cycle life compared to other lithium battery chemistries, limiting their longevity. Also noteworthy is the susceptibility of these batteries to thermal runaway events if improperly handled or subjected to adverse conditions; thus requiring robust safety mechanisms within the design of the battery system.
Looking at the Features of Lithium Cobalt Oxide batteries more closely:
- Voltages: These cells have a nominal voltage of around 3.6 volts.
- Specific Energy (Capacity): They exhibit high specific energies up around 150-200 Wh/kg.
- Charge (C-Rate) & Discharge (C-Rate): These batteries can be charged at a rate typically up to 1C and discharged at varying rates depending on the specific cell design.
- Cycle Life: The cycle life usually falls within the region of 500-1000 cycles before significant capacity loss occurs.
- Thermal Runaway: Safety concerns are inherent due to the risk of thermal runaway at elevated temperatures or if overcharged.
In terms of Applications, Lithium Cobalt Oxide cells are prevalent in personal electronics such as smartphones, tablets, laptops, and digital cameras owing to their compact size and substantial energy containment properties.
Understanding these characteristics offers insights into why certain devices favor Lithium Cobalt Oxide for power storage solutions despite its limitations in safety and cycle durability. Keeping an eye on both strengths and weaknesses is pivotal when designing systems that incorporate this type of lithium battery technology.
Pros Of Lithium Cobalt Oxide and Cons of Lithium Cobalt Oxide
Pros of Lithium Cobalt Oxide (LiCoO2) | Cons of Lithium Cobalt Oxide (LiCoO2) |
---|---|
High Energy Density: Stores a significant amount of energy relative to size. | Low Thermal Stability: Increased risk of overheating and thermal runaway. |
Stable Voltage Output: Provides consistent power delivery for optimal functionality. | Diminished Cycle Life: Lower longevity with frequent charging and discharging. |
Mature Technology: Well-established with a wealth of production and safety data. | Limited Load Capabilities: Not optimized for high-rate discharge applications. |
Fast Charge Capabilities: Allows for quick replenishment of energy levels. | Material Costs: Cobalt is expensive and less abundant, raising overall costs. |
Sleek Aesthetics: Can be produced in sleek and slim formats without sacrificing capacity. | Ethical Sourcing Concerns: Cobalt extraction raises ethical concerns. |
Environmental Impact: Recycling processes for cobalt and by-products present challenges. |
This table outlines the pros and cons of Lithium Cobalt Oxide (LiCoO2) batteries, emphasizing their high energy density, stable voltage output, mature technology, fast charge capabilities, and aesthetic appeal, while addressing concerns related to thermal stability, cycle life, load capabilities, material costs, ethical sourcing, and environmental impact.
Features Of Lithium Cobalt Oxide
Lithium Cobalt Oxide (LiCoO2) batteries are renowned for their high energy density, which is a significant factor in their widespread use in portable electronic devices such as smartphones and laptops. The voltage of a typical lithium cobalt oxide cell stands at around 3.6 volts, which contributes to the higher potential energy available in compact applications.
When examining the specific energy or capacity, these batteries are among the leaders, providing approximately 150-200 Wh/kg. This makes them highly suitable for applications where weight and space savings are critical. Charging capabilities, often referred to by their C-rate, can be moderately fast; however, they typically do not support the ultra-fast charging that some newer chemistries offer. The discharge rate is similarly moderate and well-suited for steady power delivery over extended periods.
The cycle life of lithium cobalt oxide batteries tends to be shorter compared to other lithium-based technologies, with an expectancy ranging from about 500 to 1,000 cycles before significant capacity degradation occurs. One of the concerns with LiCoO2 chemistry is thermal runaway—a condition where the battery overheats and can potentially lead to fires or explosions if damaged or improperly charged. Therefore, stringent safety mechanisms are integral when utilizing these cells.
Applications of lithium cobalt oxide span across various consumer electronics due to its ability to provide strong energy outputs while maintaining a slim form factor—a key consideration in design aesthetics and functionality in today’s mobile devices. Despite competition from other types of lithium batteries boasting improved lifecycles or safety features, LiCoO2 remains a cornerstone of high-energy portable power solutions.
Lithium Manganese Oxide
Lithium Manganese Oxide, denoted as LiMn2O4 or LMO, is a type of lithium battery chemistry that has gained recognition for its blend of stability and efficiency. The primary advantages of Lithium Manganese Oxide batteries stem from their high thermal threshold and inherent safety features. These batteries exhibit a notable resilience to overheating, which reduces the risk of thermal runaway—a critical situation where the battery could ignite due to excessive heat. With a moderate specific energy capacity, LMO batteries offer satisfactory performance for applications that require a reliable power source without excessively heavy usage demands.
However, there are constraints associated with Lithium Manganese Oxide batteries that must be acknowledged. Their life cycle tends to be more limited compared to other lithium battery types—that is, the number of complete charge and discharge cycles they can undergo before noticeable degradation occurs is lower. In addition to this, the energy density in LMO batteries typically falls short in comparison with alternatives like Lithium Cobalt Oxide or Lithium Nickel Cobalt Aluminium Oxide cells. This translates into bulkier batteries for the same amount of energy storage, which can be disadvantageous in space-critical applications.
In terms of technical specifications, Lithium Manganese Oxide cells commonly operate at voltages around 3.7-4.0 V. The specific energy (capacity) hovers around 100-150 Wh/kg which is suitable for light-duty tasks but less ideal for extended-use scenarios requiring higher capacities. When considering charge rates (C-rate), LMO batteries support moderate charging speeds but might not sustain the very rapid charge rates characteristic of some contemporary cell technologies. Conversely, their discharge rates are relatively robust and well-suited to power electronics that demand bursts of high power consumption within short periods.
Renowned for their long-standing usability in the realm of consumer electronics such as mobile phones and digital cameras, LMO batteries have also secured roles in medical devices and industrial grade equipment where operational safety takes precedence over capacity demands.
Overall, while offering some key benefits like enhanced safety profiles and adequate power delivery capabilities suitable for various devices needing instantaneous power supply rather than continuous high-energy output over long durations, Lithium Manganese Oxide cells have certain limitations regarding energy density and life span among existing lithium-ion battery assortments available on today’s market.
Pros of Lithium Manganese Oxide and Cons of Lithium Manganese Oxide
Pros of Lithium Manganese Oxide (LiMn2O4 or LMO) | Cons of Lithium Manganese Oxide (LiMn2O4 or LMO) |
---|---|
High Thermal Stability: Enhanced safety under stress or overheating. | Lower Energy Density: Stores less energy per unit of weight. |
Environmental Benignity: Manganese is more abundant and less toxic. | Shorter Lifespan: Faster capacity fade over time and with cycling. |
High Power Output: Suitable for applications requiring rapid discharge rates. | Performance at Higher Temperatures: Decline in performance and safety risks. |
Cost-Effective: Generally less expensive due to the lower cost of manganese. | Voltage Limitations: Delivers a lower nominal voltage than some alternatives. |
Safety Concerns at Higher Temperatures: Thermal management may add complexity. |
This table outlines the pros and cons of Lithium Manganese Oxide (LiMn2O4 or LMO) batteries, highlighting their high thermal stability, environmental benignity, high power output, and cost-effectiveness, while addressing limitations related to lower energy density, shorter lifespan, performance at higher temperatures, voltage constraints, and safety concerns.
Features Of Lithium Manganese Oxide
Lithium Manganese Oxide batteries, or LiMn2O4, are known for their high safety profile and long-lasting cycle life. They operate effectively within a voltage range typically around 3.7 to 4.2 volts. The specific energy or capacity of these batteries is modest, often ranging between 100-150 Wh/kg, making them suitable for applications where energy density is not the primary concern.
The charging rate (C-rate) for Lithium Manganese Oxide is moderate; these batteries can be charged at a rate that typically does not exceed 0.7C to 1C, which means it would take about an hour or slightly more to charge them fully. For discharging, they handle rates well up to around 1C-2C without significant performance degradation, allowing the energy stored to be released steadily and safely.
In terms of cycle life, these batteries deliver commendable durability with typically over 300-700 full discharge cycles before reaching end-of-life criteria. This longevity makes them attractive for applications requiring frequent charging and discharging.
One of the pivotal features of Lithium Manganese Oxide is its thermal stability which reduces the chances of thermal runaway – a condition where temperatures escalate to the point causing potential combustion. This aspect contributes significantly to its reputation as a safer lithium battery option.
Regarding applications, Lithium Manganese Oxide batteries are advantageous in scenarios that value safety above energy density — such as medical devices, power tools, and certain electric vehicles where the risk associated with thermal events must be minimal. These batteries are also used in combination with other lithium-ion materials to enhance overall battery performance.
Lithium Nickel Manganese Cobalt Oxide
Lithium Nickel Manganese Cobalt Oxide, often referred to as NMC, comprises several lithium battery types widely utilized in various applications. A balanced chemistry of nickel, manganese, and cobalt in this type of battery offers a harmonious blend of safety, energy density, and cost-effectiveness.
Pros Of Lithium Nickel Manganese Cobalt Oxide and Cons Of Lithium Nickel Manganese Cobalt Oxide
Pros of Lithium Nickel Manganese Cobalt Oxide (NMC) Batteries | Cons of Lithium Nickel Manganese Cobalt Oxide (NMC) Batteries |
---|---|
High Energy Density: Offers higher energy density compared to Lithium Iron Phosphate (LFP). | Lower Thermal Stability: Reduced thermal stability may lead to safety concerns under extreme conditions. |
Balanced Characteristics: Provides a blend of energy density, power capability, and longevity. | Cobalt Content Issues: Ethical sourcing concerns and supply chain uncertainties due to cobalt scarcity. |
Versatility: Adaptable for either energy or power, suitable for various applications. | Capacity Fade: May experience pronounced reduction in capacity over time, affecting long-term performance. |
Low Internal Resistance: Allows for faster charging and discharging rates. | Performance Variability: Different NMC versions with varying nickel, manganese, and cobalt ratios impact performance. |
Safety Margins: Thermal stability contributes to safety across consumer and industrial applications. | Environmental Concerns: Lifecycle issues regarding mining impacts, challenges with recycling, and cobalt sourcing. |
This table outlines the pros and cons of Lithium Nickel Manganese Cobalt Oxide (NMC) batteries, highlighting their high energy density, balanced characteristics, versatility, low internal resistance, and safety margins, while addressing limitations related to lower thermal stability, cobalt content issues, capacity fade, performance variability, and environmental concerns.
Features Of Lithium Nickel Manganese Cobalt Oxide
Lithium Nickel Manganese Cobalt Oxide (NMC) batteries are recognized for their versatile performance and are widely used across various applications. When considering the features of NMC, it is important to closely examine several key aspects.
Voltages: NMC cells typically operate at a nominal voltage of around 3.6V-3.7V, providing an excellent balance between energy density and safety.
Specific Energy (Capacity): This type of lithium battery has a relatively high specific energy, often in the range of 150-220 Wh/kg, making it suitable for applications where weight and space are constraints.
Charge (C-Rate): The charge rate for NMC batteries varies depending on the manufacturer and specific chemistry but generally supports fast charging capabilities. Typical values might be around 1C to 3C, enabling quicker recharge times without significant degradation in battery life.
Discharge (C-Rate): NMC batteries can typically discharge at rates around 2C-5C, which means they can support applications requiring bursts of high power output compared to some other types of lithium batteries.
Cycle Life: The cycle life reflects a cell’s longevity before its capacity drops to a certain level of the original capacity. For NMC batteries, this is typically in the range of 1000-2000 cycles under normal operating conditions which makes them quite durable over time with proper management.
Thermal Runaway: One area that requires attention with NMC batteries is thermal management; they can be susceptible to thermal runaway if not properly handled or if subjected to harsh conditions like overcharging or high temperature environments. Advanced Battery Management Systems (BMS) are often necessary to ensure safe operation.
Applications: Thanks in part to their balanced properties, NMC lithium batteries are deployed in a spectrum of applications including electric vehicles (EVs), portable electronics like laptops and smartphones, medical devices, power tools, and even grid storage solutions due to their reliable performance profile.
Overall, the versatility offered by Lithium Nickel Manganese Cobalt Oxide batteries positions them as an attractive option for many modern-day electronic needs where efficiency and reliability are paramount.
Lithium Nickel Cobalt Aluminium Oxide
Lithium Nickel Cobalt Aluminium Oxide, often abbreviated as NCA, is a type of lithium-based battery that integrates nickel, cobalt, and aluminum into its cathode. NCA batteries are recognized for their high energy density and long life cycle which makes them a suitable choice for various applications including electric vehicles and portable electronics.
The pros of Lithium Nickel Cobalt Aluminium Oxide batteries include an impressive specific energy capacity, which allows these batteries to store more energy per unit weight compared to many other types of lithium batteries. This characteristic results in extended run times for devices and can contribute to longer ranges in electric vehicles.
Conversely, the cons associated with NCA technology typically stem from cost considerations and thermal stability concerns. The materials used in these batteries are relatively expensive, which can drive up manufacturing costs. Furthermore, while NCA batteries include some inherent safety features there is still a susceptibility to thermal runaway if the battery is damaged or improperly managed.
Examining the features of Lithium Nickel Cobalt Aluminium Oxide further:
- Voltages: The nominal voltage for an NCA cell usually stands around 3.6 volts.
- Specific Energy (Capacity): These cells exhibit one of the highest capacities available today with typical specific energies ranging from 200 Wh/kg to almost 300 Wh/kg.
- Charge (C-Rate) / Discharge (C-Rate): They support moderate charging rates; however excessive charging speeds may impair battery longevity.
- Cycle Life: One of the benefits of NCA technology is its long cycle life but it is slightly overshadowed by some other chemistries like Lithium Iron Phosphate (LFP).
- Thermal Runaway: While less prone than some other lithium-ion batteries, NCA cells still require stringent monitoring systems due to concerns over thermal events.
- Applications: Owing to their high energy density and cycle stability, you will find these cells frequently used in power tools, e-bikes, EVs as well as stationary energy storage systems where space and weight efficiency are crucial.
While offering a balance between performance metrics such as capacity and durability these cells necessitate careful handling to maintain operational safety and longevity.
Pros of Lithium Nickel Cobalt Aluminium Oxide and Cons Of Lithium Nickel Cobalt Aluminium Oxide
Pros of Lithium Nickel Cobalt Aluminium Oxide (NCA) Batteries | Cons of Lithium Nickel Cobalt Aluminium Oxide (NCA) Batteries |
---|---|
High Specific Energy: Offers higher energy density, suitable for weight-sensitive applications. | Thermal Runaway Risk: Potential risk of thermal runaway, requiring meticulous battery management for safety. |
Efficient Charging: Impressive charge rates, facilitating quick recharging in fast-paced scenarios. | Shorter Cycle Life: Tends to have a shorter cycle life compared to some other lithium battery chemistries. |
Respectable Lifespan: Maintains capacity over numerous charge cycles, providing reliable energy. | Higher Production Costs: Complex chemistry may lead to higher production expenses compared to simpler variants. |
Enhanced Safety: Incrementally improved thermal stability with the inclusion of aluminium in the cathode. | Sensitivity to Conditions: Increased sensitivity to high temperatures and improper handling, requiring strict adherence to guidelines. |
Peak Power Capabilities: Can deliver significant bursts of energy, beneficial for high-power demanding applications. | Limitations in Robustness: Constraints may limit suitability for uses prioritizing robustness over energy density. |
This table outlines the pros and cons of Lithium Nickel Cobalt Aluminium Oxide (NCA) batteries, highlighting their high specific energy, efficient charging, respectable lifespan, enhanced safety, and peak power capabilities. It also addresses limitations related to the potential risk of thermal runaway, shorter cycle life, higher production costs, and sensitivity to conditions that may impact robustness.
Features Of Lithium Nickel Cobalt Aluminium Oxide
Lithium Nickel Cobalt Aluminium Oxide (NCA) batteries are known for their exceptional performance in terms of energy density and life cycle. In terms of voltages, NCA cells typically operate at an average voltage of around 3.6-3.7 volts, which is advantageous for applications requiring a high voltage platform.
The specific energy, or capacity, is another strong point of NCA batteries; they can deliver upwards of 200 Wh/kg, making them a heavyweight contender when it comes to storing a significant amount of energy in a relatively compact package. As for charge and discharge rates (C-Rate), NCA batteries maintain a good balance between rapid charging abilities and sustaining high levels of discharge without compromising the battery’s integrity.
Our focus on the cycle life reveals that NCA batteries can endure hundreds to thousands of charge-discharge cycles while retaining high functionality. This robust cycle life translates into longevity and reliability over time, mitigating the need for frequent replacements.
Addressing thermal runaway risks is critical with any battery chemistry. For NCA batteries, while they are generally safe under proper usage conditions, they demand meticulous attention to temperature management systems due to their higher energy densities. They may be prone to overheating if not properly cooled or if charged/discharged outside manufacturer specifications.
Applications leveraging the strengths of lithium nickel cobalt aluminium oxide include electric vehicles, portable electronics, and power tools where the demands for extended runtimes and battery longevity intersect—attracting industries seeking a blend of durability and energy capacity.
Lithium Titanate
Lithium Titanate (LTO) batteries distinguish themselves from other lithium technologies through their notable stability and fast charging capabilities. Pros of Lithium Titanate include an exceptional cycle life, often exceeding 7,000 cycles, which makes them a highly durable option compared to the standard lithium-ion counterparts. They also charge more rapidly; some cells can charge to 80% in under 30 minutes. Moreover, LTO batteries are less prone to thermal runaway events, enhancing safety in applications where this is a critical factor.
On the cons side, LTO batteries offer a lower specific energy (capacity), usually around 60–80 Wh/kg, which is significantly less than that provided by other lithium battery types. This can limit their use in applications where energy density is key, such as for long-range electric vehicles.
The features of Lithium Titanate reflect its robustness and efficiency. These batteries operate at voltages typically around 2.4V per cell. When it comes to specific energy (capacity), they are on the lower end of the spectrum but compensate with a high charge (C-rate) and discharge (C-rate). The ability to sustain rapid charging and discharging still renders them suitable for certain uses where quick energy transfer is more important than storage capacity.
In terms of cycle life, LTO batteries excel; their durability translates into an extended operational lifespan, reducing replacement frequency and cost over time for users. Additionally, these batteries maintain better performance at low temperatures compared to other lithium varieties.
Applications include emergency power supplies where quick charging is vital or industrial applications that need frequent and rapid charging cycles without compromising battery longevity—such as automated guided vehicles or electric buses in urban public transport systems. Despite their lower energy density, LTOs’ safety profile enables integration into advanced energy storage systems that demand stable power delivery with minimal risk of thermal incidents.
Pros of Lithium Titanate and Cons Of Lithium Titanate
Pros of Lithium Titanate Batteries | Cons of Lithium Titanate Batteries |
---|---|
Exceptional Cycle Life: Endures thousands of charge-discharge cycles with minimal degradation. | Lower Specific Energy: May not hold as much charge for its size, requiring larger and heavier batteries. |
Rapid Charge Capability: Can be charged at high C-rates, achieving near-full charge in minutes. | Higher Cost-Per-Kilowatt-Hour: More expensive compared to other lithium battery types in terms of energy density. |
Enhanced Safety: Reduced risk of thermal runaway, making them generally safer under adverse conditions. | Initial Higher Purchase Price: Upfront costs can be a barrier for adoption in certain applications. |
Performance at Low Temperatures: Retains functional capacity in cold climates. | Lower Efficiency at Lower Voltage: Less efficient in converting stored energy back into power in certain scenarios. |
Low-Voltage Design: Prolongs the lifespan of electronic components by minimizing stress. | Challenges in Market Availability: Less common, potentially leading to challenges in finding compatible infrastructure and replacement parts. |
This table outlines the pros and cons of Lithium Titanate batteries, emphasizing their exceptional cycle life, rapid charge capability, enhanced safety, performance at low temperatures, and low-voltage design. It also addresses drawbacks such as lower specific energy, higher cost-per-kilowatt-hour, initial higher purchase price, lower efficiency at lower voltage, and challenges in market availability.
Features Of Lithium Titanate
Lithium Titanate batteries distinguish themselves through a set of unique characteristics. Their voltage profile typically operates at a lower range, with cells providing around 2.4 to 2.9 volts. This is in contrast to other lithium-ion batteries that usually operate at higher voltages. Despite this, lithium titanate stands out for its specific energy or capacity, which is typically lower, falling in the range of 30-110 Wh/kg.
A prominent advantage is their impressive charge (C-rate) and discharge (C-rate) capabilities. Lithium titanate can be fast-charged, often within minutes rather than hours, and offers high rate discharge performance without suffering significant degradation, which is crucial for applications requiring immediate bursts of power.
When it comes to cycle life, lithium titanate excels dramatically over traditional lithium-ion counterparts. Cells can endure upwards of 3,000-7,000 cycles before reaching end-of-life conditions—a significant increase in longevity that makes them ideal for repetitive use over extended periods.
Thermal stability and safety are also areas where lithium titanate shows superiority; they have a much-improved resistance to thermal runaway events when compared to other chemistries like Lithium Cobalt Oxide or Lithium Nickel Manganese Cobalt Oxide. As a result of these features, they operate safely across a broader temperature range.
Applications for lithium titanate encompass those demanding high cycle life and rapid charging/discharging capabilities such as electric buses and grid storage systems where dependable long-term performance is critical. However, due to their lower energy density and higher costs relative to alternative technologies like nickel-based systems or more common lithium-ion chemistries, their use has been specialized rather than widespread within the consumer electronics sector.
What’s the Most Common Type of Lithium Battery?
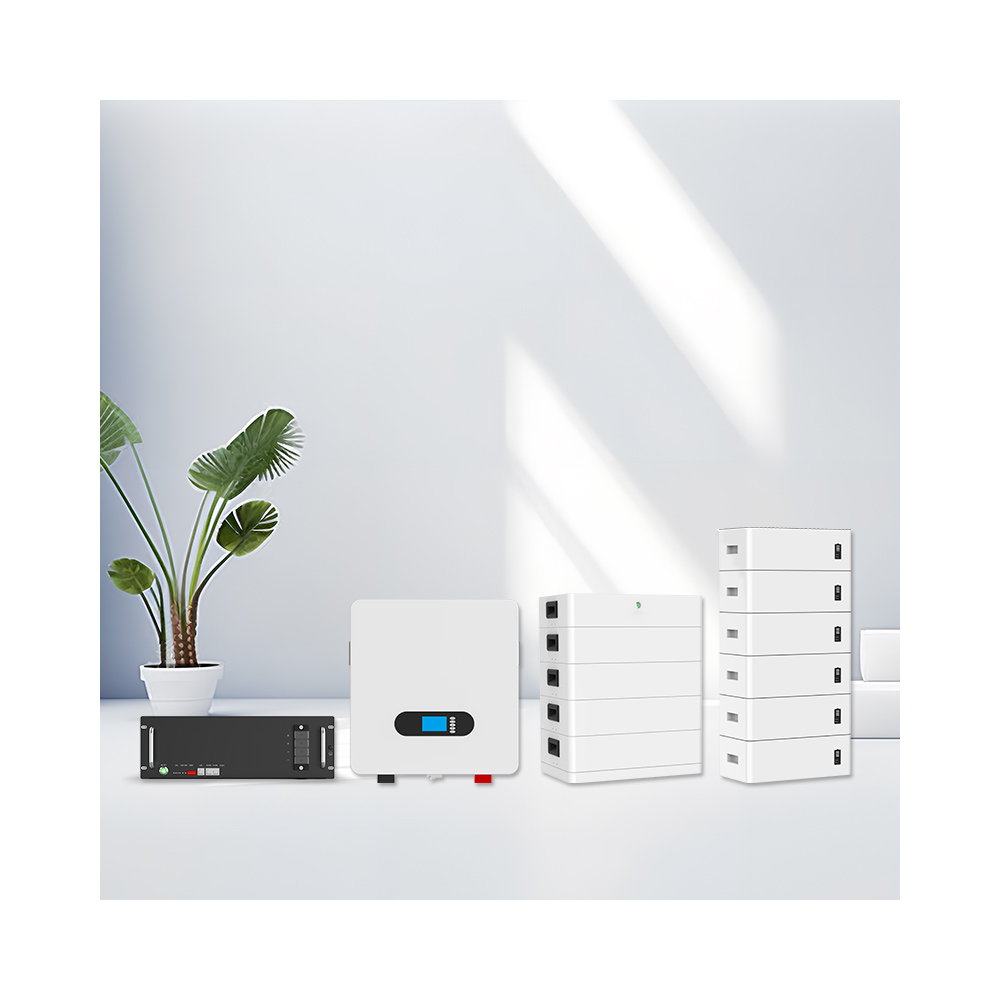
The most widespread type of lithium battery in use today is the Lithium-Ion (Li-ion) battery, specifically the Lithium Cobalt Oxide variant. This particular chemistry dominates the mobile electronics market due to its high energy density, which enables longer usage between charges—a pivotal feature for devices like smartphones, laptops, and tablets. Its prevalence is attributed to its early development and commercialization, which helped establish a strong foothold in consumer electronics. The ability to hold more charge per unit of weight makes Lithium Cobalt Oxide batteries particularly suitable for applications where weight and space are critical constraints.
Despite newer lithium battery technologies emerging with potentially superior performance and safety profiles, Lithium Cobalt Oxide remains the most familiar due to its established manufacturing processes and extensive deployment over decades. As other types develop regarding stability, cost-effectiveness, and energy capabilities, they may challenge the dominance of Lithium Cobalt Oxide batteries in various market segments; yet as of current standings, this type retains the title for most common usage across a broad spectrum of electronic devices.
Do All Types Of Batteries Use Lithium?
Contrary to what the presence of many lithium-based batteries in the market might suggest, not all types of batteries use lithium. Lithium is an important element in battery technology due to its high electrochemical potential which lends itself to creating lightweight, high-energy-density batteries. However, there are various other kinds of battery chemistries that do not incorporate lithium. These include lead-acid batteries, commonly found in automotive starters; nickel-cadmium (NiCd) and nickel-metal hydride (NiMH) batteries, which have applications from portable electronics to hybrid vehicles; and sodium-sulfur or flow batteries for grid energy storage solutions.
Among these alternatives, lead-acid batteries are notable for their reliability and lower cost but suffer from a lower energy density. NiCd batteries offer robust performance with a significant degree of durability but have fallen out of favor due to environmental concerns over cadmium. NiMH technology provides a more environmentally friendly option with a better energy density than NiCd and is particularly popular in hybrid electric vehicles.
In high-demand sectors such as electric mobility and large-scale energy storage systems, lithium-ion variants spearhead the advancements due to their superior characteristics in terms of energy density and cycle life. However, it is clear that while lithium-based batteries are prevalent especially for applications where weight and volume need minimization, they do not represent the entirety of available battery technologies on the market today.
The diversity of battery types ensures that various needs across different sectors can be met effectively. It also illustrates ongoing innovation within the field as researchers continue to seek alternatives that may offer benefits like reduced costs or improved safety profiles compared to existing lithium-based options.
What Makes A Good Battery For Energy Storage Systems
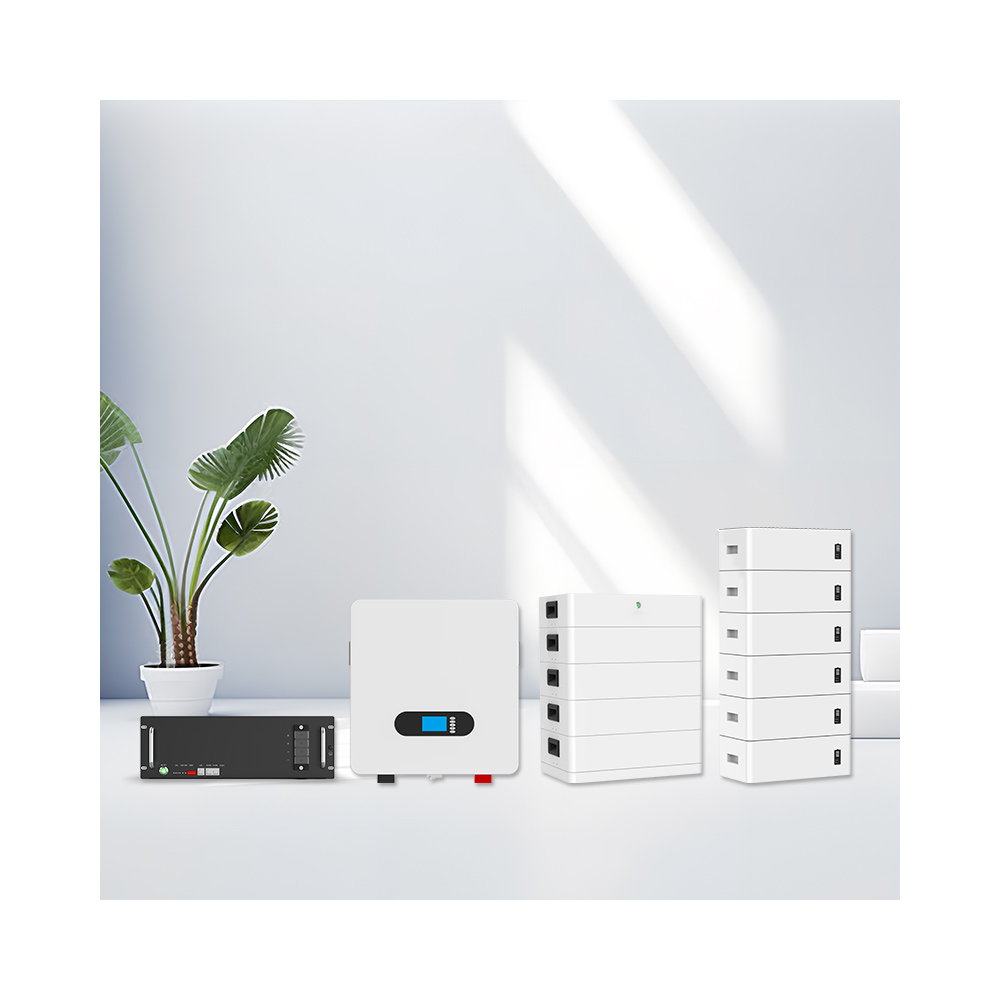
When assessing the adequacy of batteries for energy storage systems, several key characteristics become vital. A good battery should exhibit a high number of cycles, indicating longevity and cost-effectiveness over time. Batteries with more cycles are preferred for energy storage because they can endure many rounds of charging and discharging without significant degradation.
High peak power is another crucial attribute. This denotes the ability of a battery to deliver substantial power output when needed, even if only for short periods. Batteries capable of high peak power are essential in applications where an instant surge of energy is necessary.
Low production cost contributes to economic feasibility, making large-scale deployment and adoption of energy storage solutions more achievable. Batteries that can be produced at lower costs while maintaining performance standards are key to integrable and scalable energy systems.
To ensure safety and reliability, minimising chances of thermal runaway events is essential. Thermal runaway is a dangerous condition in some battery chemistries where an increase in temperature changes the conditions in a way that causes a further increase in temperature, often leading to catastrophic failures or fires. A battery design that inherently reduces the risk or severity of thermal runaway adds an important layer of security to its use, encouraging wider acceptance among users.
In summary, those developing batteries for energy storage systems strive for these attributes – longevity through many charge cycles, capability for high peak power output, affordability in mass production, and intrinsic safety features against thermal runaway – as they collectively frame what makes a good battery platform not only viable but also competitive for such applications.
In Conclusion
In summary, the diverse range of lithium batteries, from Lithium-ion to Lithium Iron Phosphate, offers a variety of options tailored for specific needs and applications across multiple industries.
Discover the optimal power solution for your requirements by further exploring our extensive selection of lithium batteries. Contact us today to learn more about how these advanced battery types can enhance the efficiency and performance of your devices or systems.